Sulfur-based species induced photochromism in sodalite
Keywords: Hackmanite, Tenebrescence, Photochromism
Sodalite Na4 (Al3 Si3 O12)Cl is a natural aluminosilicate mineral that contains sulfur or sulfur-related defects, known as chromophores (Reinen et al. 1999; Fleet et al. 2010). Hackmanite, a variety of sodalite, exhibits unusual optical properties such as photoluminescence, tenebrescence, or photochromism upon absorption of UV light, causing a change in color that can be reversed (see Fig. 1) (Williams et al. 2010). Since Kirk’s research on the photochromism of sodalite in 1955, the cause of the photochromism of hackmanite has been studied. It has been attributed to a sulfur-related defect and, more recently, an interaction between disulfide ions and chloride vacancies (Kirk 1955, Agamah 2020). However, the exact mechanism is still under investigation (Blumentritt 2021). The objective of this study is to examine the role of sulfur-related defects on the photochromism of hackmanite by investigating the spectroscopic properties of sodalite and hackmanite.
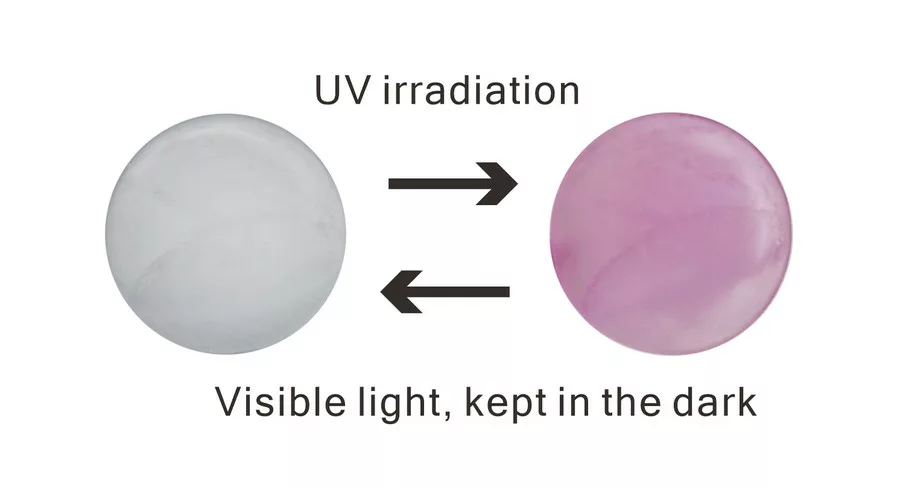
Thirteen stones used in this study were cabochon-cut from Myanmar, comprising eight hackmanites with color ranges of very pale yellow, light gray, and red hues, and five sodalites with blue hues. The stones weighed between 0.45 and 1.67 carats and ranged in transparency from translucent to semi-opaque (see Fig. 2).
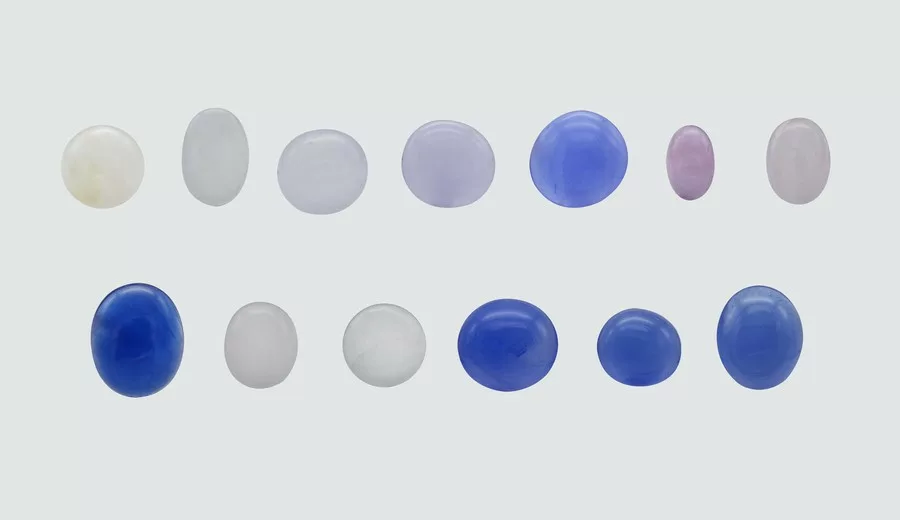
UV-Vis spectra were obtained using a JASCO UV-Vis spectrometer (V-660, Japan) with a bandwidth of 2 nm. The transmission mode spectrum was measured in the range of 200 to 800 nm at a scan rate of 400 nm per minute. Chemical analyses were performed using Energy-Dispersive X-ray Fluorescence spectroscopy (EDX-8000, Shimadzu, Japan) with an acceleration voltage of 50 kV and a beam current of 1000 µA measured by irradiating the sample using a 1000 µm collimator. Additionally, X-ray photoelectron spectroscopy (XPS) was used to determine the oxidation state of sulfur, using a Nexsa instrument from ThermoFisherScientific.
Table 1 displays the results of the chemical analysis conducted on the 13 stones. The weight percentages of SiO2 and Al2 O3 , which form the basic framework of both sodalite and hackmanite, were found to be similar, as expected. As per Jackson’s definition, hackmanite is identified as a sulfur-bearing sodalite that exhibits fluorescence (Jackson 1997). The photochromism of hackmanite is believed to be associated with the presence of sulfur (Agamah 2020, Carvalho 2018). However, sulfur was detected in all samples at levels lower than approximately 0.4 wt.%, with the highest concentration being found in the sodalite sample. XPS analysis revealed that SO4 2- ions were present in all samples, while mono-/polysulfur species were not detected.

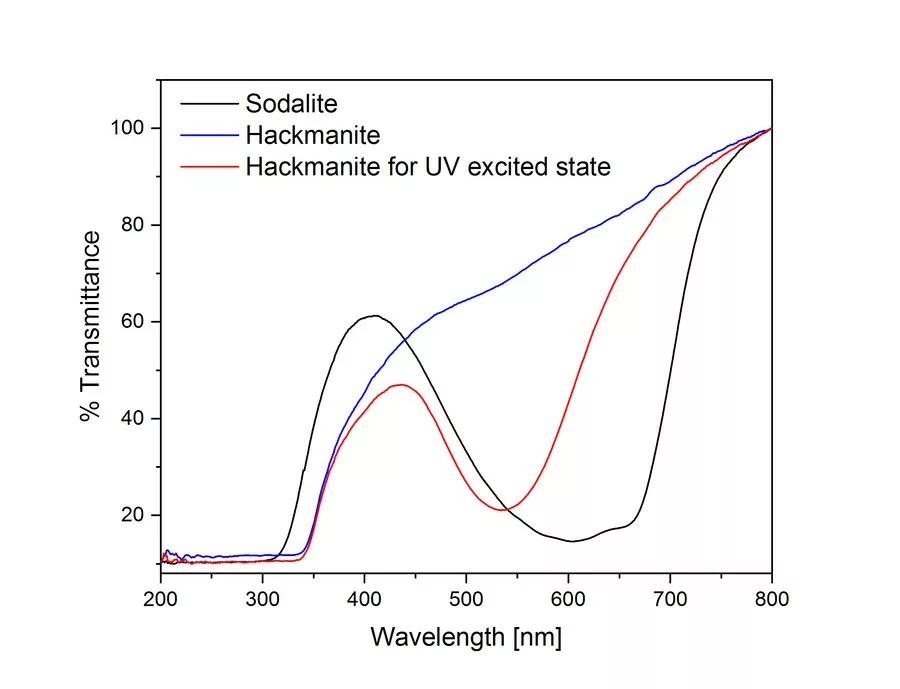
The optical properties of sodalite and hackmanite are clearly shown in the UV-Vis spectra (Fig. 3). In previous studies (Williams et al. 2010; Stoliaroff et al. 2021), the origin of color in hackmanite is that one of electrons of the disulfide ion is captured at a chlorine vacancy and acts as a color center (F-center). Such charge transfer requires energy corresponding to the ultraviolet region (Medved 1953).
A blue spectrum was measured before hackmanite was exposed to UV light, and a red spectrum was measured after exposure (using a Fabel FUV-R2 lamp with a wavelength of 365 nm) in Figure 3. An absorption band due to the chlorine vacancy is observed over a broad range centered at 530 nm. This absorption decreases with time, which can take from minutes to hours depending on the stone, in a dark room or under visible light and returns to the pre-UV irradiation state (as shown in Fig. 2).
The UV-Vis spectrum of blue sodalite (Figure 3) was found to be identical before and after UV irradiation, indicating the absence of photochromism. The blue color of sodalite is known to be caused by tri-sulfur radical ions substituted for the chlorine site, resulting in an absorption band at 595 nm (Reinen et al., 1999). However, in this study, the blue sodalite sample showed a combined spectrum of two absorption bands centered at 600 nm and 660 nm, suggesting the existence of different cage environments containing trisulfide or other types of sulfides.
Based on these measurements, it can be summarized that the manifestation of photochromism is not proportional to the concentration of sulfur and is likely due to the presence of trace sulfur-related defects that meet certain conditions, such as disulfide ions and chloride vacancies.
References:
- Agamah, C., et al., 2020. Hackmanite—The Natural Glow-inthe-Dark Material, Chemistry of Materials, 32, 8895-8905.
- Blumentritt, F., Fritsch, E., 2021. Photochromism and Photochromic Gems: A Review and Some New Data (Part 1), The Journal of Gemmology, 37(8), 780–800.
- Carvalho, J.M., Norrbo, I., Ando, R.A., Brito, H.F., Fantini, M.C.A. & Lastusaari, M., 2018. Fast, low-cost preparation of hackmanite minerals with reversible photochromic behavior using a microwave-assisted structure conversion method. Chemical Communications, 54(53), 7326–7329.
- Fleet, M.E., Liu, X., 2010. X-Ray Absorption Spectroscopy of Ultramarine Pigments: A New Analytical Method for the Polysulfide Radical Anion S3- Chromophore, Spectrochimica Acta, Part B, 65 (1), 75-79.
- Jackson, J., 1997. Glossary of Geology, 4th ed., American Geological Institute, Alexandria, VA, 288.
- Kirk, R.D., 1955. The luminescence and tenebrescence of natural and synthetic sodalite, American Mineralogist, 40 (1-2), 22-31.
- Kondo, D., Beaton, D., 2009. Hackmanite/Sodalite from Myanmar and Afghanistan, Gems & gemology, 45 (1), 38-43.
- Medved, D.B., 1953. The Optical Properties of Natural and Synthetic Hackmanite, Journal of Chemical Physics, 21 (7), 1309-1310.
- Reinen, D., Lindner, G.-G., 1999. The nature of the chalcogen colour centres in ultramarine-type solids. Chemical Society Reviews, 28 (2), 75-84.
- Stoliaroff, A., Schira, R., Blumentritt, F., Fritsch, E., Jobic, S., Latouche, C., 2021. Point Defects Modeling Explains Multiple Sulfur Species in Sulfur-Doped Na4 (Al3 Si3 O12)Cl Sodalite, The Journal of Physical Chemistry C, 125 (30), 16674-16680.
- Williams, E., Simmonds, A., Armstrong, J., Weller, M., 2010. Compositional and structural control of tenebrescence, Journal of Materials Chemistry, 20, 10883-10887.