Irradiation treatment and gamma-spectroscopy of morganite
The pink variety of beryl, morganite, is one of the most popular orangey-pink to pink gemstones. Its colour is attributed to manganese, both Mn2+ and Mn3+ (Nassau & Wood, 1968, Wood and Nassau, 1968, Schmetzer et al., 1974, Shigley and Ford, 1984, Deer et al., 1992), which produce a series of spin-allowed absorption bands between 450 to 600 nm. Their exact position and quantity depends on the strength and symmetry of the local crystal field, which, among others, also depends on the substitution mechanisms on the Be-position in the crystal lattice (Platonov et al., 1989). By thermal enhancement, the colour of pink morganites is usually not influenced much (Schmetzer et al., 1975), however, orangey hues can be removed. By artificial irradiation the colour saturation of colourless to pale pink manganese-bearing beryl is stronglyintensified, due to an intensification of the manganese-band system (see also Schmetzer et al., 1975). Iron-bearing samples become orangey-pink to brown, due to an additional intensification of the oxygen-metal-charge-transfer-band system (OMCT-band system, Fe3+-O2 —CT). These samples can be heat treated after irradiation, weakening the OMCT-band system again and resulting in pure pink colours.
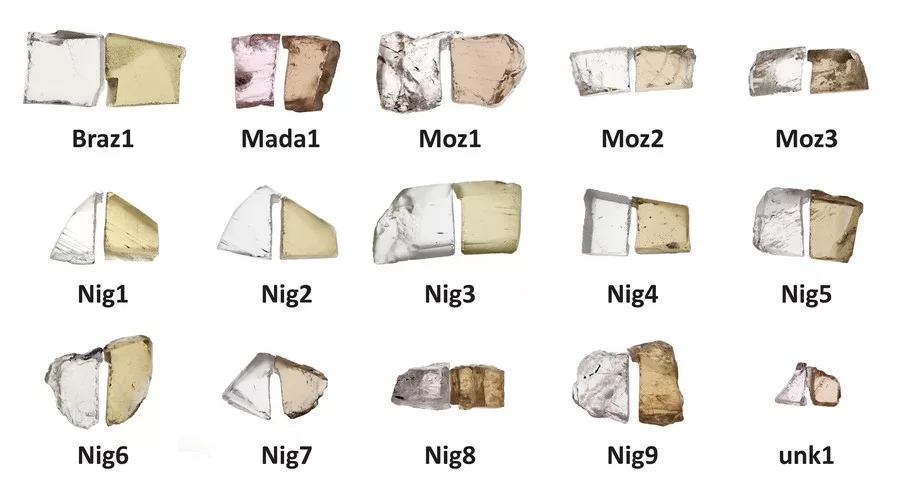
Usually by gamma irradiation, mainly the OMCT-band system of iron-bearing beryl is increased, the pink colour component (Mn) enhances just weakly to not at all. By irradiation with neutrons in nuclear reactors or accelerators also the pink colour component is intensified, however, most morganites, due to their natural caesium contents, become strongly radioactive, mainly by a production of the radioactive isotope Cs-134 from the stable isotope Cs-133 (see e.g. Kitawaki et al., 2012). Therefore, the method-of-choice for the artificial enhancement of the pink hue of morganites by irradiation nowadays is the bombardment with electrons in accelerators. Using this technique only very little Cs is activated to Cs-134, resulting in activities usually far below legal limits.
The most common instrument to detect radioactivity is the Geiger counter, which, however, just indicates if the material is radioactive, but it cannot detect the type or amount of radionuclides causing the radioactivity. Gamma-spectroscopy is used to determine the type of radioactive isotopes and their activity. A detailed description of the technique and the applications in gemmology has been published by Ashbaugh in 1992. In 2012, Kitawaki et al. used gamma-spectroscopy to detect the activation products Cs-134, Mn-54 and Zn-65 in two large morganites irradiated by neutrons. Here we provide for the first time the evidence for usefulness of gamma-spectroscopy to identify also the electron irradiation treatment.
For this study, manganese-bearing morganites from different origins have been targeted irradiated with electrons. Afterwards, the samples were measured by gamma-spectroscopy to identify the kinds and activity of the induced radioactive nuclides, mainly Cs-134, which can be seen as evidence for artificial irradiation. The samples were selected from different localities with various natural Cs-contents. The intent is to prove if there is still enough Cs-134 produced in samples with low Cs-concentration, to clearly identify the treatment. Cs-134 has a half-life time of about 2.1 years only, thus, it could not be found in the natural samples relaxed at the geological time scales and its analytical evidence should be considered as a direct hint to the artificial irradiation treatment. On the other side, because of this relative short half-life time the technique is not expected to be expedient for morganites from old stocks. However, in combination with technologies like e.g. blockchain, it could be possible to separate natural and treated morganites, from recent mining
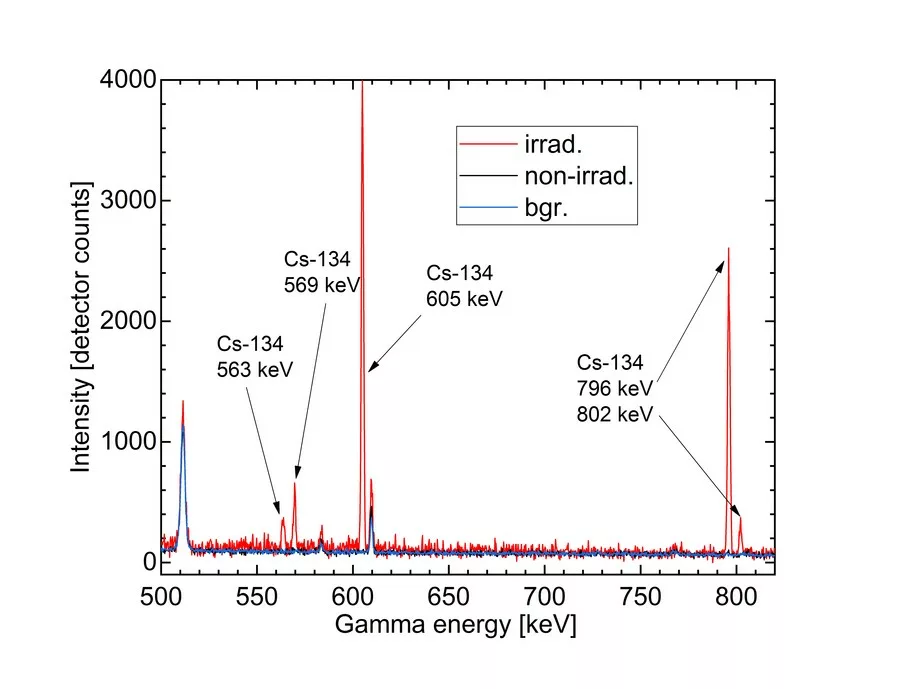
Material and methods
For this study, fifteen morganites from the reference collection of the German Gemmological Association were cut into wafers with windows parallel to the c-axis. Different origin locations (Brazil, Madagascar, Mozambique and Nigeria) were covered. Each sample was cut into two halves (Fig. 1). One half remained untreated; the other half has been irradiated with electrons using typical industrial praxis of 10 MeV energy for 6 hours. Depending on their exact position during irradiation process small differences between single samples may occur, but the overall total dose of approximately 250 MGy was reached in all of them. The samples were irradiated together with a big lot of gem-quality morganites in a proprietary irradiation facility.
Polarized optical absorption spectra were measured with a Perkin Elmer Lambda 950S UV/Vis/NIR spectrometer in the range between 200 to 2500 nm with a resolution of 1 nm. Chemical compositions have been determined with an energy dispersive X-ray fluorescence, a Thermo Scientific ARL Quant’X EDXRF. A special beryl programme has been used to calculate the oxide concentrations; BeO was fixed to 14 %, calibration was done with well-analyzed beryl reference samples.
Gamma-spectroscopy was performed at the FRM II Research Neutron Source of the Technical University in Munich using a Canberra/Mirion Technologies coaxial high-purity germanium (HPGe) semi-conductor detector (GX4018) with a relative efficiency of 40 % and an energy resolution of 1.8 keV at 1332 keV connected with a Lynx multichannel analyser. The Genie 2000 software package was used for data analysis.
Results and discussion
Due to the irradiation, all samples became yellowish-brown to orangey-brown in colour, caused by an intensification of both, the OMCT- and the Mn3+-absorption bands. Using a Gamma-Scout® radiation detector no radiation exposure has been detected. By gamma-spectroscopy, Cs-134 was identified in the irradiated halves, while none was detected in the untreated samples (Fig. 2). Moreover, in the very recently irradiated samples also Cs-132 radioisotope with half-life time of 6.8 days only could be found. It can serve as an additional marker to indicate that irradiation took place within the last 2-3 month. Due to the short half-life time of Cs-134, this technique is effective just for morganites that were recently exposed to artificial radiation. Depending on the caesium concentration of the initial material and the energy it has been exposed to, we expect that identification is possible within few half-life times depending on sample mass, Cs concentration, counting time and gamma-detector efficiency (e.g. up 8-10 years). For samples from old stocks this method may not be appropriate. In combination with techniques tracking the material from mine to market, e.g. blockchain technologies, it can be a powerful tool to separate untreated (or thermally enhanced) material from gems artificially irradiated by high energy electrons. Cs-134 as a product of the neutron capture reaction and is not expected in untreated morganites, since corresponding reactions occur in the nature extremely rarely, combined with the short half-life of Cs-134.
Outlook
We plan more detailed investigations to determine the limits of the proposed method. In a next step, the samples investigated for this report will be heated to remove the yellow colour components and reach the pink hue enhancement. No change in the gamma spectrum is expected by the heating treatment and this will be proved. The samples will be re-measured again at regular intervals over the next few years to determine the threshold Cs-concentration at which the activity of Cs-134 falls below the detection limit, but also to determine the minimal sample size and maximum detection time after irradiation.
References:
- Ashbaugh III, C. E., 1992. Gamma-ray Spectroscopy to Measure Radioactivity in Gemstones. Gems & Gemology 28 (2), 104-111.
- Deer, W. A., Howie, R. A. and Zussman, J., 1992. The rock forming minerals. Vol. I Longman Group Ltd., England.
- Kitawaki, H., Horikawa, Y., Shozugawa, K. and Nogawa, N., 2012. Radioactive Morganite. Gems & Gemology 48 (1) 42- 44.
- Nassau, K. and Wood, D. L., 1968. An examination of red beryl from Utah. Am. Mineral. 53, 801-806.
- Platonov, A. N., Taran, M. N. and Klyakhin, V. A., 1989. On two colour types of Mn3+-bearing beryls. Z. Dt. Gemmol. Ges. 38 (4), 147-154.
- Schmetzer, K., Berdesinski, W. and Bank, H., 1974. Über die Mineralart Beryll und Absorptionsspektren. Z. Dt. Gemmol. Ges. 23 (1), 5-39.
- Schmetzer, K., Berdesinski, W. and Bank, H., 1975. Farbveränderungen von Edelsteinen der Beryllgruppe. Z. Dt. Gemmol. Ges. 24 (2), 81-87.
- Shigley, J. E. and Foord, E. E., 1984. Gem quality red beryl from the Wah-Wah mountains, Utah. Gems & Gemology 20 (4), 208-221.
- Wood, D. L. and Nassau, K., 1968. The characterization of beryl by visible and infrared absorption spectroscopy. Am. Mineral. 53, 777-800.